Minimizing the Risk of Biological Specimen Mix-Up in the Mammography Unit: A Failure Mode and Effect Analysis (FMEA) Methodology in Oncology Setting
Download
Abstract
Background: Effective cancer management relies heavily on early diagnosis, which significantly improves patient outcomes by enabling timely and accurate treatment decisions. Despite advancements in diagnostic tools, the risk of specimen mix-ups remains a critical challenge, particularly in the pre-analytic phase of specimen collection.Purpose: This study aimed to minimize the risk of biological specimen mix-ups in the mammography unit in Oncology center through the implementation of the Failure Mode and Effect Analysis (FMEA) methodology.
Methods: A proactive FMEA was conducted in the mammography unit, targeting the pre-analytic phase of specimen collection. A multidisciplinary team identified potential failure modes, assessed their severity, occurrence, and detection, and calculated the Risk Priority Numbers (RPNs). Interventions were developed and implemented to address high-risk areas, with post-intervention RPNs evaluated to measure the effectiveness of the changes.
Results: The analysis revealed high-risk failure modes, including patient misidentification, labeling errors, and inadequate specimen tracking, with initial RPNs ranging from 280 to 360. Interventions such as standardizing patient identification, improving consent processes, and enhancing specimen labeling systems led to significant RPN reductions across all categories, with the most notable improvement observed in site marking (67% reduction).
Conclusion: The proactive use of FMEA effectively identified, mitigated the risks, and enhanced the safety and reliability of specimen collection in the mammography unit. This approach highlights the importance of applying systematic risk management processes in improving diagnostic accuracy and patient safety, providing a model for other healthcare settings.
Introduction
Effective cancer management is fundamentally linked to the early diagnosis of cancer, which remains one of the most critical, yet challenging aspects in the oncology field. Accurate diagnosis and timely screening are essential to improving survival rates and enabling appropriate patient treatment decisions [1-3]. Early detection allows for interventions at a stage when the cancer is most treatable, significantly enhancing patient outcomes. Globally, approximately 2.3 million women are diagnosed with breast cancer annually, making it the most prevalent cancer among women worldwide [2-3].
Early diagnosis emphasizes reducing barriers that hinder patients from accessing healthcare services promptly. This approach aims to enhance the proportion of individuals accurately diagnosed with cancer by utilizing advanced and precise diagnostic tools [4-6]. Screening for breast cancer typically begins with a mammogram, which is a low-dose X-ray capable of detecting abnormal tissue [5-7]. If irregularities are identified, further diagnostic evaluations such as diagnostic mammography, ultrasound, or additional imaging tests are conducted to confirm the findings. Depending on the results, patients may be referred to short-interval follow-up imaging or a biopsy to ensure a definitive diagnosis [8-10].
Despite advancements in diagnostic tools and methodologies, early diagnosis and screening still face significant challenges, particularly the risk of specimen mix-ups [10-13]. Specimen identification errors can compromise diagnostic accuracy, leading to potentially severe consequences for patients [13, 14]. The diagnostic process relies not only on pathologists during the analytic phase but also on the critical roles of clinical practitioners in the pre-analytic and post-analytic phases. Evidence suggests that approximately 50% to 80% of diagnostic errors arise from sampling mistakes, highlighting the importance of meticulous handling throughout the entire diagnostic process [15].
To mitigate these risks, healthcare institutions implement the Failure Mode and Effect Analysis (FMEA) method as a robust risk management tool. FMEA is designed to identify, assess, and address potential risks, thereby enhancing the quality of specimen sampling processes [15-23]. In Oncology Center in Oman, the FMEA method has been integrated into the mammography unit within the radiology and nuclear medicine department to minimize errors and prevent adverse events. This approach not only decreases operational variability but also improves diagnostic accuracy, increases efficiency, boosts production volumes, and reduces operational costs [6, 7]. During routine observations at the mammography unit, a significant operational bottleneck was identified in the specimen sampling process. To address this issue proactively, the FMEA method was employed to evaluate potential risks and streamline workflow within the mammography unit. By implementing this systematic and preventive approach, the center aims to optimize the mammography process, ensuring accurate and timely breast cancer diagnoses while maintaining high-quality standards in patient care. This study aimed to minimize the risk of biological specimen mix-up in the mammography unit through FMEA.
Methods
Setting
This project was conducted in the mammography unit of the Radiology and Nuclear Medicine Department at the Oncology Center, Muscat, Oman. This unit plays a critical role in breast cancer early detection by ensuring the accurate collection of patient specimens.
Design
The project was designed to address operational bottlenecks identified during the observation phase in the specimen sampling collection process at the mammography unit. A FMEA method was applied to systematically assess and manage potential risks. A multidisciplinary team comprising of radiographers, surgical oncologists, technicians, and quality assurance members collaborated to map the process, identify potential failure modes, and assign Risk Priority Numbers (RPN), which is calculated based on the potential failure severity, occurrence, and detectability matrix, where this RPN helps prioritize risks. Insights from the potential failures root cause analysis informed the development of targeted mitigation strategies [16-26]. The project timeline spanned from the third quarter of 2023 to the first quarter of 2024, culminating in implementing the recommendations.
FMEA methodology
Defining the Process
The FMEA began by targeting the specimen collection process within the mammography unit, a critical component of breast cancer diagnosis. The primary goal was to minimize the risk of specimen mix-ups and ensure the reliability of diagnostic results. A detailed flowchart , as described in Figure 1, was developed to visually represent the process, breaking it into distinct subprocesses and identifying key stages where risks could potentially arise.
Figure 1. Flowchart for Specimen Collection in the Mammography Unit.
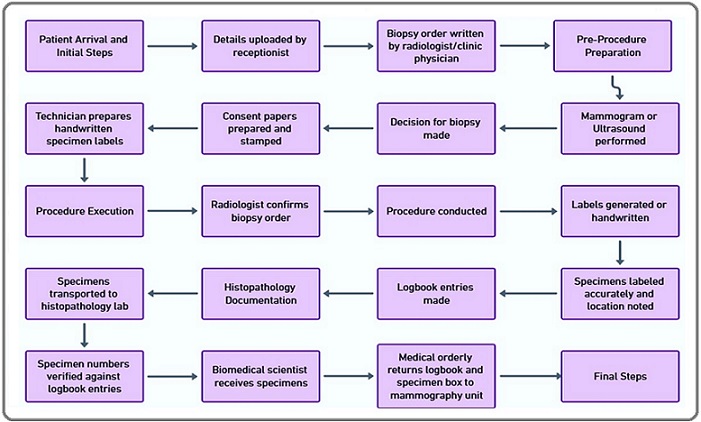
It illustrates a comprehensive mapping of the step-by-step process of specimen collection in the mammography unit, outlining key stages from patient preparation to sample labeling and transportation by providing a clear foundation for subsequent analyses and interventions.
Identify Potential Failure Modes
A multidisciplinary team, consisting of radiographers, surgical oncologists, technicians, and quality assurance members, conducted a brainstorming session to identify all possible failure modes within the specimen collection process. These failure modes included procedural errors, labeling inaccuracies, and storage mishandlings, among others. Each failure mode was further analyzed to determine its root causes and potential effects on patient safety and diagnostic accuracy. To facilitate this analysis, the team created a fishbone diagram .Please refer to Figure 2.
Figure 2. Fishbone Diagram for Risk of Biological Specimen Mix-Up.
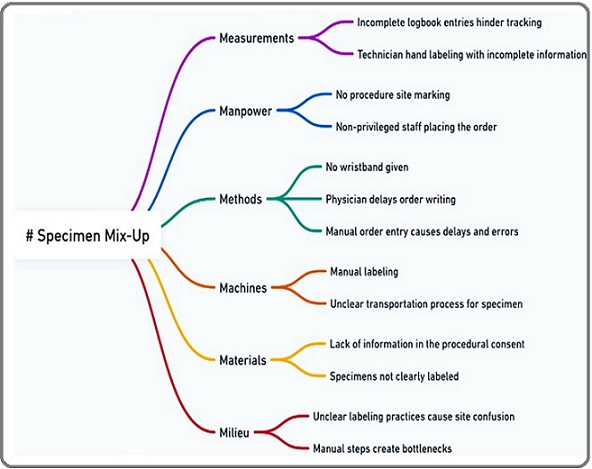
This fishbone diagram identifies potential causes of biological specimen mix-ups, categorizing contributing factors under key domains such as measurements, manpower, methods, machines, materials, and milieu. This visual tool streamlined root cause analysis and allowed for a structured approach to risk mitigation.
Evaluating the Effects of Each Failure Mode
Each failure mode identified was systematically evaluated for its potential effects on the overall process and patient outcomes. The analysis considered factors such as the impact on diagnostic timelines, patient safety, and the accuracy of test results. This step provided a deeper understanding of the consequences associated with each failure mode, helping prioritize areas for intervention.
Assigning a Severity Rating
The severity of each failure mode was rated on a scale from 1 to 10, with higher scores indicating more severe consequences for patient safety and diagnostic accuracy. For instance, a specimen mix-up leading to an incorrect diagnosis would receive a high severity rating due to its potential to result in inappropriate treatment or delayed care. This step ensured that high-impact risks were clearly identified.
Assigning a Likelihood of Occurrence Rating
The likelihood of each failure mode occurring was also rated on a scale of 1 to 10. Higher ratings reflected greater probabilities of the failure mode occurring during routine operations. For example, a lack of standardized procedures for labeling specimens might result in a high occurrence rating due to the increased potential for errors.
Assigning a Detection Rating
The ability to detect and mitigate failures before they impact patient outcomes was assessed on a scale from 1 to 10. Higher detection ratings corresponded to lower capabilities for identifying the failure mode. This step highlighted areas where the process was most vulnerable and where enhanced detection mechanisms were necessary.
Calculating RPN
The RPN was computed for each failure mode by multiplying its severity (S), occurrence (O), and detection (D) ratings. This value served as a quantitative measure to rank risks and prioritize them for corrective action. Failure modes with the highest RPNs were identified as critical issues requiring immediate attention. This prioritization guided resource allocation and focused improvement efforts on areas with the greatest potential impact.
Identifying and Implementing Corrective Actions
Based on the RPN calculations, the team developed and implemented targeted corrective actions to address the most critical risks based on previous investigations and published studies [27-39]. Strategies included revising workflows to standardize specimen handling, enhancing staff training on proper labeling and storage protocols, and introducing additional process checks at key stages. These interventions aimed to reduce the likelihood of errors and improve detection capabilities. For example, double-check procedures for specimen labeling were introduced to mitigate the risk of mix-ups. Additionally, staff training programs were conducted to reinforce the importance of adherence to protocols.
After implementing corrective actions, the team recalculated the RPNs to evaluate the effectiveness of the interventions. A reduction in RPNs indicated that the corrective measures successfully mitigated risks. This step ensured continuous improvement and validated the efficacy of the FMEA methodology in enhancing the quality and safety of the specimen collection process within the mammography unit.
Ethical consideration
The project received Institutional Review Board (IRB) approval under the reference number CCCRC-119-2024, ensuring that all research activities complied with ethical standards and safeguarded confidentiality and welfare. This approval reflects adherence to rigorous ethical guidelines, including data protection and minimal risk to participants.
Results
Initial RPN
The analysis of clinical workflows identified several critical areas of concern, focusing on failures, their causes, effects, and associated RPN. In patient identification, the absence of wristbands has previously been linked to a lack of proper policy implementation. This failure resulted in the misidentification of patients, with an RPN of 300. Additionally, improper patient identification due to insufficient training and awareness increased the risk of sample mix-ups, with an RPN of 320. Please refer to Table 1.
Process | Main Failure Modes | Causes | Effects | Initial RPN | Post-intervention RPN | Difference (%) |
Patient Identification | No wristband for patient identification | Lack of proper policy implementation | Misidentification of patients | 300 | 145 | 52 |
Patient Identification | No proper patient identification | Insufficient training and awareness | Increased risk of sample mix-up | 320 | 120 | 63 |
Consent Process | Consent paper signed by technician, not physician | Misunderstanding of consent responsibilities | Legal and ethical issues, patient safety concerns | 280 | 112 | 60 |
Procedure Labeling | Procedure details not specific (e.g., no side specified) | Lack of detail in order documentation | Confusion regarding procedure specifics, potential errors | 340 | 148 | 56 |
Specimen Labeling | Handwritten labels with incomplete information | Lack of standardized labeling process | Incorrect specimen identification | 360 | 148 | 59 |
Specimen Collection | Unclear process for specimen collection | No defined procedure for order entry | Delays and errors in specimen processing | 280 | 110 | 61 |
Histopathology Documentation | Inconsistent logbook entries | Inadequate documentation practices | Inaccurate tracking of specimens | 300 | 125 | 58 |
Site Marking | No site marking as per policy | Lack of adherence to marking policy | Increased risk of wrong site procedures | 360 | 120 | 67 |
The Consent Process previously faced issues where consent papers were signed by technicians instead of physicians. This failure, caused by misunderstandings of consent responsibilities, led to legal and ethical concerns, as well as risks to patient safety (RPN: 280). Procedure labeling suffered from insufficient detail in order documentation, such as missing site specifications. This failure created confusion regarding procedure specifics and increased the likelihood of errors, reflected by an RPN of 340. Specimen labeling was another critical area, where handwritten labels with incomplete information were common. The absence of a standardized labeling process led to incorrect specimen identification, with an RPN of 360.
The Specimen collection process had previously been hindered by unclear procedures for order entry. This lack of defined protocols caused delays and errors in specimen processing, resulting in an RPN of 280. In Histopathology documentation, inconsistent logbook entries, driven by inadequate documentation practices, led to inaccuracies in tracking specimens. This failure was associated with an RPN of 300. Lastly, Site marking was found to lack adherence to marking policies, resulting in a failure mode where no site marking was performed. This significantly increased the risk of wrong-site procedures, with an RPN of 360.
Interventions
The interventions outlined addressed critical risks across several clinical processes to enhance safety, accuracy, and efficiency based on previous studies in the field [27-39]. For patient identification, improving practices and enforcing identification policies were key to eliminating the risk of patient misidentification. In the consenting process, clarity and compliance were reinforced by involving the physician in obtaining patient consent, ensuring adherence to legal and ethical standards. Similarly, procedure documentation was standardized to ensure accuracy and reduce ambiguity, minimizing potential errors in clinical workflows (Table 2).
Category | Intervention |
Patient Identification | Enhance patient identification practices and enforce the patient identification policy |
Consenting Process | Improve the consenting process by ensuring clarity and compliance through the involvement of the physician performing the procedure in obtaining patient consent |
Procedure Documentation | Standardize procedure documentation for accuracy and completeness |
Specimen Labeling and Handling | Adopt a standardized approach for specimen labeling and secure handling. |
Histopathology Documentation | Improve histopathology practices with robust specimen-tracking systems |
Site Marking and Verification | Improve site marking procedures with thorough verification steps and enforce the safe surgery policy |
Order Verification and Security | Strengthen order verification and implement security measures |
For specimen labeling and handling, a standardized approach was implemented to prevent mix-ups, ensuring the secure handling and accurate identification of specimens. Histopathology was enhanced with robust tracking system to improve specimen documentation and processing accuracy. To mitigate surgical risks, site marking and verification procedures were refined, incorporating thorough verification steps and adherence to safe surgery policies. Finally, order verification and security measures were strengthened to prevent unauthorized access and enhance order processing reliability. These interventions collectively addressed systemic weaknesses, improving overall patient safety and operational effectiveness.
Post RPN Post-intervention
The data demonstrates significant improvements in RPN across all processes after implementing targeted interventions, highlighting enhanced safety and efficiency. For patient identification, the initial RPN for the absence of wristbands dropped from 300 to 145, and the RPN for improper identification decreased from 320 to 120. These reductions indicate that enforcing identification policies and enhancing staff training effectively mitigated the risks of patient misidentification and sampling mix-ups. Please refer to Table 1.
In the consent process, the RPN dropped from 280 to 112 by ensuring that physicians, rather than technicians, obtained informed consent, addressing legal and ethical concerns. Similarly, for procedure labeling, standardizing order documentation reduced the RPN from 340 to 148, minimizing confusion and potential errors. Also, specimen labeling showed a notable improvement, with a reduction of RPN from 360 to 148, reflecting the impact of standardizing the labeling processes.
Further, in specimen collection, implementing clear procedures for order entry reduced the RPN from 280 to 110, improving processing accuracy. For histopathology documentation, the RPN dropped from 300 to 125 due to enhanced logbook practices and tracking systems. Lastly, for site marking, adherence to marking policies reduced the RPN from 360 to 120, significantly lowering the risk of wrong-site procedures. Collectively, these improvements demonstrate the effectiveness of the implemented interventions in addressing high-risk areas and enhancing clinical process safety.
Figure 3 depicts the reduction in RPN following the implementation of corrective interventions.
Figure 3. Percentage of Improvement in RPN after Interventions.
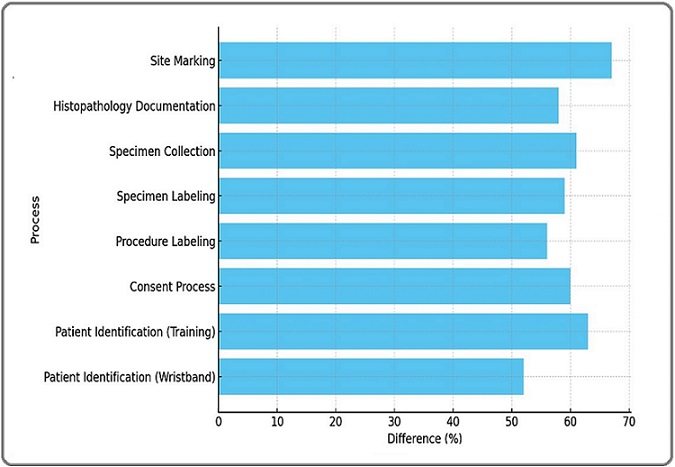
The comparison highlights the impact of process improvements in minimizing specimen handling errors and enhancing patient safety. The most notable improvement was observed in site marking, which achieved a 67% reduction in RPN, reflecting enhanced adherence to marking policies. Other processes, such as patient identification, and specimen labeling, showed impressive improvements of 63% and 59%, respectively, due to improved training and standardization measures. The consenting process and specimen collection, also, achieved substantial reductions of 60% and 61%, respectively, emphasizing the effectiveness of systematic procedural refinements. These improvements demonstrated the success of interventions in enhancing clinical safety and minimizing errors.
Discussion
The primary goal of this study was to proactively assess and mitigate risks in the pre-analytic phase of the specimen collection process in the mammography unit. This initiative sought to minimize the risk of specimen mix-ups for women undergoing breast cancer diagnosis, ensuring accurate and timely treatment. Given that laboratory testing underpins approximately 60% of clinical decision-making and that 70% of errors occur in the pre-analytic phase, this study focused on addressing specific vulnerabilities such as patient identification errors, specimen misidentification, mislabeling, and inadequate specimen tracking. By adopting a proactive approach, the study aimed to create a replicable model for improving diagnostic accuracy and patient safety through systematic risk management [16-18].
The FMEA played a central role in identifying high- risk failure modes. These included inappropriate patient identification, which posed a significant risk of specimen mix-up, and errors in specimen labeling due to the absence of a standardized labeling system [19, 20]. Other critical issues highlighted by the FMEA included improper consenting procedures, inadequate documentation, unclear site marking protocols, and inconsistent histopathology tracking. Based on RPN, specimen labeling and site marking emerged as the most critical risks, given their direct impact on diagnostic accuracy and patient safety. Prioritizing these failure modes allowed the study to target the most pressing vulnerabilities effectively [22, 23].
To address these identified vulnerabilities, a series of targeted interventions were implemented. For example, errors in patient identification were addressed by introducing standardized wristbands, which eliminated inconsistencies and improved the accuracy of patient identification throughout the diagnostic workflow [26-28]. Furthermore, training programs were introduced to enhance staff awareness and adherence to protocols, reducing the risk of errors related to manual practices. Similarly, inconsistencies in the consenting process were resolved by ensuring physician involvement, reinforcing ethical and legal compliance, and streamlining procedural accuracy. Standardizing documentation, also, improved the clarity and reliability of information, reducing errors caused by ambiguity [31-33].
The interventions produced significant improvements in the reliability and safety of the pre-analytic phase. Standardized labeling systems replaced handwritten labels with pre-printed barcodes, creating a more consistent and traceable process. This innovation minimized human error and improved team coordination, ensuring that specimens were tracked accurately throughout the workflow. Verification protocols further strengthened the accuracy of specimen handling by introducing multiple checkpoints, reducing the likelihood of errors that could compromise diagnostic outcomes. These systematic improvements directly contributed to enhanced diagnostic reliability and improved patient outcomes [34-36].
Enhancements in site marking and the consent process also contributed significantly to improved results. By enforcing strict verification steps, site marking errors were minimized, reducing the risk of wrong-site procedures [36-38]. Requiring physician involvement in the consent process clarified roles and responsibilities, ensuring ethical and legal standards were upheld. These interventions not only reduced errors but also fostered a culture of accountability and precision among staff, reinforcing the mportance of a patient-centered approach to diagnostic care [31, 38, 39]. Together, these measures ensured procedural compliance and created a safer, more reliable diagnostic process [16].
The success of these interventions underscores the value of using FMEA as a framework for identifying and addressing systemic vulnerabilities. Quantifying risks through RPN scores allowed the team to focus resources on the most critical areas, while the structured nature of the methodology facilitated continuous monitoring and improvement [6, 16]. Follow-up evaluations demonstrated the sustainability of the improvements, with consistently low RPN scores across targeted processes. This outcome highlights the effectiveness of a proactive, data-driven approach to risk management in healthcare [16-20].
By addressing pre-analytic risks systematically, this study demonstrates the importance of moving beyond reactive approaches to error management. Proactive interventions not only prevent potential errors but also improve overall workflow efficiency and diagnostic reliability [17-20]. The findings validate the role of patient-centered risk management strategies in achieving sustainable improvements in clinical processes, ensuring that diagnostic care is both accurate and timely. Ultimately, the study highlights the potential for broader applications of FMEA to enhance patient safety and healthcare outcomes across different settings [20-23].
The outcomes of this study serve as a model for other healthcare institutions seeking to improve their diagnostic processes. The proactive risk assessment methodology and targeted interventions used here provide a replicable framework for addressing vulnerabilities in other departments or institutions. This discussion underscores the critical role of systematic risk management in driving sustainable improvements in patient safety and diagnostic accuracy.
Limitations of the Study
Despite its success, the study had several limitations. It focused solely on the mammography unit and did not address risks in other departments that might face similar challenges. Additionally, the study relied heavily on staff compliance, and its effectiveness could vary in settings with different levels of resource availability or staff training. The proactive approach, also required significant time and effort for planning and implementation, which could be a limitation for institutions with resource constraints.
Future Implications
The success of this initiative offers a model for other healthcare institutions seeking to enhance diagnostic accuracy and patient safety through systematic risk management. Future studies could expand this approach to other departments or institutions, focusing on integrating advanced technologies such as artificial intelligence for risk detection and process optimization. Additionally, longitudinal studies could evaluate the long-term sustainability of the interventions and their broader impact on healthcare outcomes.
In conclusion, this study highlights the effectiveness of the proactive FMEA methodology in addressing pre-analytic phase risks and improving patient safety. By systematically identifying vulnerabilities and implementing targeted interventions, the study achieved significant improvements in diagnostic accuracy and process reliability. The findings demonstrate the importance of proactive risk assessment in establishing a culture of safety and accountability in healthcare settings.
Acknowledgments
The authors acknowledge the support of the Sultan Qaboos Comprehensive Cancer Care and Research Center, particularly the Radiology and Nuclear Medicine Department. The success of this project would not have been possible without the dedicated efforts of the multidisciplinary team, including radiographers, oncologists, technicians, and quality assurance staff. Their collaboration and commitment to patient safety were instrumental in achieving the study’s objectives.
Conflict of Interest
Author declares no conflict of interest
References
- New mammography screening performance metrics based on the entire screening episode Sprague BL , Miglioretti DL , Lee CI , Perry H, Tosteson AAN , Kerlikowske K. Cancer.2020;126(14). CrossRef
- Quality in cancer diagnosis Raab SS , Grzybicki DM . CA: a cancer journal for clinicians.2010;60(3). CrossRef
- Breast Cancer-Epidemiology, Risk Factors, Classification, Prognostic Markers, and Current Treatment Strategies-An Updated Review Łukasiewicz S, Czeczelewski M, Forma A, Baj J, Sitarz R, Stanisławek A. Cancers.2021;13(17). CrossRef
- Performance comparison of different machine learning models in breast cancer diagnosis. New Generation Technologies and Sustainability in Healthcare Uzun FN . 2024.
- SMART Hybrid Models for Improved Breast Cancer Detection. Proceedings on Advances in AI-based Biomedical Imaging Terlapu PV , Gali NR , Mandavakuriti Y. 2024.
- Breast cancer prediction using machine learning classification algorithms. Intelligence-Based Medicine Almustafa KM , La Moglia A. 2025.
- Understanding cellular proliferation activity in breast cancer using multi-compartment model of transverse relaxation time mapping on 3T MRI Nkonde KA , Cheung SM , Senn N, He J. Frontiers in Oncology.2025;15. CrossRef
- Expression and Subtype Discordance Between Core Needle Biopsy and Surgical Specimen in Breast Cancer Xu M, Yan Y, Chen Y, Chen X, Gong K, Fu F. The Journal of Surgical Research.2025;307. CrossRef
- Evaluating the performance of artificial intelligence and radiologists' accuracy in breast cancer detection in screening mammography across breast densities Caldas FAA , Caldas HC , Henrique T. Eur J Radiol.2025.
- Clinical utility of tumor-infiltrating lymphocyte evaluation by two different methods in breast cancer patients treated with neoadjuvant chemotherapy Nagahashi M, Ishikawa E, Nagai T, Kanaoka H, Oshiro A, Togashi Y, Hattori A, et al . Breast Cancer (Tokyo, Japan).2025;32(2). CrossRef
- Common errors in radiology leading to missed breast cancer diagnosis: Recommendations from EUSOBI Thomassin-Naggara I, Kilburn-Toppin F. Eur Radiol.2024.
- Reasons for false-negative and false-positive diagnosis in fine needle aspiration cytology of the breast: 286 cases with cyto-histopathologic correlation Barut F, Bahadir B. Turkish J Pathol.2025.
- Improving Breast Cancer Diagnosis through Advanced Image Analysis and Neural Network Classifications Kanagamalliga S, Dandu Bhavya V. Procedia Computer Science.2025;252. CrossRef
- Bounding boxes for weakly-supervised breast cancer segmentation in DCE-MRI Zhong Y, Xu Z, Han C, Liu Z, Wang Y. Biomedical Signal Processing and Control.2025;105. CrossRef
- Micro-computed Tomography in the Evaluation of Eosin-stained Axillary Lymph Node Biopsies of Females Diagnosed with Breast Cancer Laguna-Castro S, Salminen A, Arponen O, Hannula M, Rinta-Kiikka I, Hyttinen J, Tolonen T. Scientific Reports.2024;14(1). CrossRef
- Risk Assessment-Oriented Design of a Needle Insertion Robotic System for Non-Resectable Liver Tumors Gherman B, Hajjar NA , Tucan P, Radu C, Vaida C, Mois E, Burz A, Pisla D. Healthcare (Basel, Switzerland).2022;10(2). CrossRef
- FMEA of MR-Only Treatment Planning in the Pelvis Kim J, Miller B, Siddiqui MS , Movsas B, Glide-Hurst C. Advances in Radiation Oncology.2019;4(1). CrossRef
- How to apply clinical cases and medical literature in the framework of a modified "failure mode and effects analysis" as a clinical reasoning tool--an illustration using the human biliary system Wong KC . Journal of Medical Case Reports.2016;10. CrossRef
- Skin biopsy: Identifying and overcoming errors in the skin biopsy pathway Stratman EJ , Elston DM , Miller SJ . Journal of the American Academy of Dermatology.2016;74(1). CrossRef
- Radiology failure mode and effect analysis: what is it? Abujudeh HH , Kaewlai R. Radiology.2009;252(2). CrossRef
- Using Failure Mode and Effects Analysis in Improving Nursing Blood Sampling at an International Specialized Cancer Center Haroun A, Al-Ruzzieh MA , Hussien N, Masa'ad A, Hassoneh R, Abu Alrub G, Ayaad O. Asian Pacific journal of cancer prevention: APJCP.2021;22(4). CrossRef
- Evaluating the application of failure mode and effects analysis technique in hospital wards: a systematic review Asgari Dastjerdi H, Khorasani E, Yarmohammadian MH , Ahmadzade MS . Journal of Injury & Violence Research.2017;9(1). CrossRef
- Minimizing the Risk of Sample Mix-ups in the Molecular Pathology Section in Oncology Center Using Risk Assessment Matrix (RAM) Al Haddabi IH , Ibrahim R, AlSheidi SA , Busaidi A, Ghufran N, AlDhahli SN , et al . ResearchGate.2025;10(1):37-45. CrossRef
- Evaluating radiotherapy treatment delay using Failure Mode and Effects Analysis (FMEA) Xu Z, Lee S, Albani D, Dobbins D, Ellis RJ , Biswas T, Machtay M, Podder TK . Radiotherapy and Oncology: Journal of the European Society for Therapeutic Radiology and Oncology.2019;137. CrossRef
- Risk analysis and safety assessment in surgical robotics: a case study on a biopsy robot Korb W., Kornfeld M., Birkfellner W., Boesecke R., Figl M., Fuerst M., Kettenbach J., et al . Minimally invasive therapy & allied technologies: MITAT: official journal of the Society for Minimally Invasive Therapy.2005;14(1). CrossRef
- Enhancing patient safety: optimizing fall risk management for oncology patients through Failure Modes and Effects Analysis Majed M, Ayaad O, AlHasni NS , Ibrahim R, AlHarthy SH , Hassan KK , et al . Asian Pac J Cancer Prev.2024;25(2):689.
- Optimizing Laboratory Processes: A Path to Reduced Sample Rejection in Oncology AlSheidi SA , Ayaad O, Ibrahim R, AlDhahli SM , Majed M, AlWaheibi HM , Zadjali ROAA , et al . Iranian Journal of Public Health.2025;54(1). CrossRef
- Examining Nurse and Patient Factors Before and After Implementing an Oncology Acuity Tool: A Mixed Methods Study Al-Ruzzieh MA , Eddin R, Ayaad O, Kharabsheh M, Al-Abdallah D. Journal of Nursing Measurement.2024;32(1). CrossRef
- Challenges with the management of young women’s breast cancer in Gulf countries and the Middle East Al Ajmi AA , Ayaad O, Al Aghbari S, Al Balushi MN , Koziha EK , Al-Ishaq Z, et al . J Young Womens Breast Cancer Health.2024;1(1-2):36-41.
- Improving diagnosis for patient safety in an oncology setting: quality initiatives Al-Baimani K, Ayaad O, Ibrahim R, Zadjali RA , Faliti BA , AlAwaisi H, et al . Sobraj Publishing Service.2024.
- Referral Process Enhancement: Innovative Approaches and Best Practices AlHarthy SH , Al-MoundhrI M, Al-Mahmoodi W, Ibrahim R, Ayaad O, Al Baimani K. Asian Pacific journal of cancer prevention: APJCP.2024;25(5). CrossRef
- Improving Care Continuity in Oncology Settings: A Lean Management Approach to Minimize Discharges Without Follow-Up Appointments AlHarthy SH , Ayaad O, Al Mashari AAA , AlBalushi MA , Ibrahim R, Bait Nasib MH , Al Zadjali R, Awaisi H, Al Baimani K. Asian Pacific journal of cancer prevention: APJCP.2024;25(4). CrossRef
- The role of total quality management in improving patient experiences and outcomes Haroun A, Ayaad O O, Al-Ruzzieh MA , Ayyad M. Br J Healthc Manag.2024;28(10):1-8.
- Improving Nurses’ Hand-off Process on Oncology Setting Using Lean Management Principles Ayaad O, Haroun A, Yaseen R, Thiab F, Al-Rawashdeh K, Mohammad I, Aqtash M, et al . Asian Pacific journal of cancer prevention: APJCP.2019;20(5). CrossRef
- Adopting Lean Management in Quality of Services, Cost Containment, and Time Management Ayaad O, Al-Dewiri R, Kasht L, Qaddumi B, Ayyad M. Asian Pacific journal of cancer prevention: APJCP.2022;23(8). CrossRef
- Organisational citizenship behaviour in the healthcare workplace: a scoping review Al-Ruzzieh MA , Al Rifai A, Ayaad O. Br J Healthc Manag.2022;28(6):1-7.
- Nursing Professional Practice Model: Development, Implementation, and Evaluation at an International Specialized Cancer Center Al-Ruzzieh MA , Ayaad O. The Journal of Nursing Administration.2020;50(11). CrossRef
- Predicting and classifying the perceptions of learning needs importance in cancer patients; a machine learning approach Ayaad O, Ibrahim R, AlBaimani K, AlGhaithi MM , Sawaya ZG , AlHasni NS , et al . Health Educ Health Promot.2024;12(4):649-660.
- Measuring Occupational Fatigue among Higher and Middle Management at a Specialized Cancer Center during the COVID-19 Pandemic Al-Ruzzieh MA , Ayaad O. Asian Pacific journal of cancer prevention: APJCP.2022;23(10). CrossRef
License

This work is licensed under a Creative Commons Attribution-NonCommercial 4.0 International License.
Copyright
© Asian Pacific Journal of Cancer Biology , 2025
Author Details